Why does Mars lack glacial landscapes?
This project was undertaken as part of a Marie Skłodowska-Curie Individual Fellowship, Horizon 2020 in 2021.
The surface of Mars is incised by thousands of valleys, evidence that the red planet once harbored surface liquid water and an active hydrological cycle. These valleys date from the Noachian period ~3.9-3.5 Byr ago, signifying that Mars’s surface harbored water, at least sporadically, at the time life first emerged on Earth. This climate optimum finished around ~3.5-3 Gyr ago, marking the beginning of Mars’ Hesperian period and the rise of a global hyperarid cryosphere, which nowadays characterizes the red planet. Polar caps, glaciers, buried ice sheets, mantling ice and dust deposits, ground ice, and permafrost now define the surface and near surface water inventory of Mars, where liquid water is no longer stable. However, the transition between the Noachian climate optimum and the current global cryosphere poses a significant geological question mark: why is the surface of Mars mostly devoid of glacial landforms? Indeed, liquid water should have remained stable for a long period of time under building glaciers and ice sheets, and there is evidence for liquid water action related to permafrost and ground ice. But landforms characteristic of water-glacier interactions such as moraines, drumlins, mega-scale glacial lineations, or polished bedrock, some of which may be very large and occupy large extensions of ground, are largely missing from Mars’ geological record. This has led scientists to believe that martian glaciers and ice sheets never hosted water underneath, rendering them unable to erode or transport material, or even that glacier and ice sheets never existed on the first place.
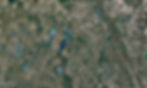
Glacial landscapes of northern Canada, Victoria Island. Notice the grooved, lineated surfaces showing the direction of ice flow. These types of landscape are largely missing on Mars.
However, work on the dynamics of terrestrial warm-based glaciers and ice sheets revealed a feedback mechanism involving glacial driving stresses and glacial sliding velocity. Lower surface gravity on Mars would hinder the driving stress, but how this would affect glacial sliding, and therefore the erosion and scour power of glaciers on the surface of Mars had not been studied. The main objective of this project was to determine whether Martian glacial masses produce a different geological fingerprint from terrestrial ones. This discrepancy would imply that Martian ancient ice sheets harbored basal water, counter to current thinking. This has large implications for our search for water and habitable environments on ancient Mars.
Building understanding of this question will help us better comprehend the geological history of Mars from its surface landforms, and understand whether water remained stable under glacial masses after the collapse of Mars’ early climate. This opens the possibility of a much longer period of time in which liquid water, and life, could have existed on the red planet. Incidentally, better understanding the formation of glacial landforms and its physical link to the internal dynamics and water availability under glaciers and ice sheets, as envisioned in this project, is key to advance our own interpretation of former terrestrial glaciations, their extent, and meltwater source and drainage dynamics. Because geomorphology is often the only key to the history of expansion and retreat of the Pleistocene ice sheets and glaciers, studying it greatly contributes to improving predictions to how the remaining large ice masses on Earth (Greenland, Antarctica, polar caps and glaciers) will evolve under a warming climate.
The objective of this project was divided in three:
(O1) Understanding the link between glacial landforms and underlying glacial dynamics, to comprehend why certain glacial landscapes on Earth also do not display 'characteristic' landforms of warm based glaciation.
(O2) Utilize the insight from O1 to explain the dynamics of warm-based glaciers on Mars with a focus on glacial hydrology, and draw insight as to why certain glacial landforms may be missing.
(O3) Explore the role of martian glaciation through time under the perspective of ice dynamics.
To proceed with objective 1, I interrogated terrestrial glaciers and ice sheets, and the origin of the landforms characteristic of warm-based glaciation, which occurs when water is present under ice. Interestingly, terrestrial glacial landscapes are vastly different depending on the characteristics of the former glacial cover: the size and shape, the surface ice slope, the amount of water under the ice, the basal topography, and the total mass. Alpine glaciation, characterized by high mountain ranges with steep slopes, left behind U-shaped valleys, subglacial gorges, moraine-dammed lakes, and moraines among others. Ice sheet glaciation is characterized by large swaths of mega-scale glacial lineations, vast terminal moraines, drumlins, polished bedrock, scouring in striations and grooves where water basal availability was large and ice sliding velocity was fast. Where glacial sliding was slower, water availability was less or an efficient drainage network existed, landforms associated to ice sheets tend to reflect their subglacial drainage structures through eskers, subglacial channels, tunnel valleys, glaciofluvial corridors, and the lower sliding velocity through ribbed moraines, or seemingly uneroded spots. Polar caps, characterized by much thinner and colder ice with only seasonal input of basal meltwater, may be missing entirely any fingerprints of warm-based glaciation. The result from this first objective is the realization that glacial drainage and the speed of glacial sliding play a key role on the final geomorphology produced by ice cover. Arguments that Mars' lack of certain landforms, such as moraines or drumlins, point unequivocally at the lack of existence of martian warm-based glaciation are thus unfounded, and likely the result of an observation bias focused on those glacial landscapes that are easily accessible, and which witnessed large, fast-sliding ice sheets without efficient drainage systems emplaced.
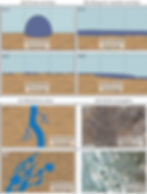
Glacial drainage and landscapes associated to different types of water subglacial flow.
To proceed with objective 2, I explored the sliding and drainage dynamics that would exist under glaciers and ice sheets on Mars, under lower surface gravity. I utilized state-of-the-art numerical models to describe terrestrial glacial hydrology, and coupled the dynamics of glacial sliding with the dominant subglacial drainage mechanism: cavities and channels. Glacial sliding, the responsible for areal glacial scouring and transport, and the deposition of moraines, builds upon largely inefficient subglacial drainage. In this mode, cavities under the glacier or ice sheet build up water, which cannot drain and therefore becomes trapped and pressurized under the ice. Effective pressure, the difference between ice overburden pressure and subglacial water, tends to zero so that partial, localized lift of the ice may occur. Basal meltwater at high pressure lubricates the glacial base, driving and accelerating glacial sliding over the base. Fast sliding speeds, large driving stresses, and the lack of an efficient drainage system favor in turn an acceleration of glacial sliding. On the other hand, the existence of an efficient drainage system greatly reduces subglacial water residence time and pressurization. Water flows within subglacial channel networks or large tunnel valleys, resembling an overpressurized river system. Sediment transported within subglacial channels may be transported towards the ice margin, producing ice terminal deltas or fans, sandurs, or may clog the subglacial conduit and produce an esker ridge upon ice retreat. Large pressures and discharges may lead to flooding, and the emplacement of glaciofluvial hummocky corridors covering tens or hundreds of kilometers in extension. Smaller sliding velocities, smaller driving stresses, and the existence of efficient subglacial drainage modes reduce glacial sliding, and may prevent the formation of lineations, glacial scouring, and moraines, as observed on the Canadian Arctic Archipelago. When interrogating the effect of the lower martian gravity on the dominant subglacial drainage mode, and thus the relative importance of glacial sliding, I found that inefficient drainage would have been strongly inhibited on Mars, in favor of the emergence of efficient networks of subglacial drainage conduits. The fingerprints of glaciation on Mars should be eskers and subglacial channel networks, whereas scouring, moraines, and glacial sliding related landforms should be rare and limited to regions with high slopes or very large water accumulations. Results from objectives 1 and 2 were published in GRL under the title 'Valley networks and the record of glaciation on ancient Mars' in 2022.
Objective 3 was accomplished in two parts, targetting the early Mars (Noachian and Hesperian epochs 4-3 Gyr before present (BP)) and late Mars period (Amazonian, 3Gyr BP to present). First, I reviewed the work done to this date on early Mars ancient glaciations, exploring ice sheet and glacial landforms formed in the past Noachian Hesperian epochs of Mars, as well as their climate and hydrological implications, and connection with subsurface water reservoirs. This included the southern circumpolar ice cap, which was formerly much more extensive than the current polar deposits, and which had regions with basal presence of water (regionally warm-based). The geomorphological unit associated, named ‘Dorsa Argentea’ displays eskers that are often much larger than many terrestrial counterparts. To this date no remaining moraines, glacial grooves or lineations, or polished bedrock have been identified in association with the southern circumpolar ancient glaciation, although evidence for former subglacial drainage in the form of corridors, meltwater ponds, possible kettle lakes, and eskers have been extensively noted. This lack of glacial scouring related landforms is coherent with the results of objective 2, stating that glacial sliding would have been largely inhibited on Mars, and shows how warm-based glaciation may have produced landscapes reflecting the former glacial drainage pathways rather than glacial scouring. Other landscapes, belonging to the boundary region between Mars’ large south-north topographic asymmetry, or belonging to the high elevation Tharsis volcanic province were also discussed, as well as the context of Mars’ ancient glaciation in terms of global climate conditions and total water inventory. This work is accepted for publications as a chapter in ‘Ices in the Solar System’, titled ‘Ice on Noachian and Hesperian Mars: Atmospheric, Surface, and Subsurface Processes’ and will be published by Elsevier in 2023.
Part two of objective 3 aimed at linking glacial ice dynamics and internal structure to the geomorphology of Amazonian ice deposits on Mars, aged around 1 Gyr BP. For this work I benefited from my participation in the Color and Stereo Surface Imaging System (CaSSIS) mission, on board the Trace Gas Orbiter under ESA’s ExoMars project, to acquire and process high resolution (4.6 m/pixel) color images of deforming debris-covered glacial deposits on Mars. I considered a Lobate Debris Apron (LDA), a type of debris-covered remnant glacial deposit found in the martian mid-latitudes, located in the northeast of the massive Hellas impact basin. This LDA presented banding patterns in its surface, alternating in color, as well as a terraced surface morphology visible in high resolution, CTX elevation data (18 m/pixel). The investigation here considered only the deformation of ice under cold-based conditions (that is, no water present at the base of the deposit), and explored the effect of orbital-induced climatic oscillations on the flow dynamics and surface morphology of the deposit. Internal structure, whether massive crystalline ice or layered ice and debris, was discussed, and its effects on ice rheology were introduced, but not yet analyzed in detail. This work was published as part of the 2022 CaSSIS Special Issue in Planetary and Space Science, titled ‘Patterns of martian glacial deformation: Implications for glacio-geology,internal structure, and regional climate’.

CaSSIS image MY34_005511_224_0_RGB showing the surface deformation patterns of a glacier located on the tropical band on Mars (around 30S)
My work opens the door to an understanding of martian glacial landscapes that is physics-based, and not solely based on direct comparison with terrestrial glacial landscapes. The dynamics of glacial flow are complex, very sensitive on temperature and stress variations, and depend also on internal structure. Therefore, it is reasonable to expect that martian landforms and landscapes produced by ice flowing at low temperatures and low driving stresses cannot be fully represented by visual comparisons with terrestrial highly dynamic and relatively warm glaciers and ice sheets. My work thus adds a basis to understand how martian glacial landscapes could be, how are they different from terrestrial, and why.
Expected results until the end of the project. Most results have already been obtained, and the main objective has been explored during the course of this project. One last publication remains to be submitted, targeting Amazonian (<1 Gyr BP) warm-based glaciation on Mars, exploring the landscapes and landforms that a regional ice cover with presence of basal water produced on a cratered region. A collaborative publication, exploring the formation of valley networks carved by meltwater originating from the subsurface, from fluidized ejecta, or from unidentified glacial sources, is currently in review. I expect the final publication of both results to be finalized by 2024.
Potential impacts. The timing of water stability on the surface of Mars has long been constrained to the so-called early Mars period, 3.9-3.5 Gyr BP. This is the time when surface liquid water was at least sporadically stable on the surface of Mars, and when large part of the development of valley networks, crater lakes, deltas, hydrated minerals, and hydrothermal activity occurred. Beyond this optimal period, and notably after 3 Gyr BP, Mars lost the bulk of its atmosphere to space and surface liquid water was no longer stable. However, multiple observations of recent landforms formed by the activity of water under glaciers, as well as many observations of recent valley systems, all dating <3 Gyr BP, indicate that water environments could have persisted much later in Mars’ history, with important astrobiological implications, locations where life could have originated or persisted through the rise of Mars’ current global hyperarid cryosphere. The impact of my work is in recognizing these environments where water could have been stable under martian ice masses, by recognizing what type of landforms and landsystems are distinctive of such environments on Mars, and how these differ to distinctive landforms of similar environments on Earth. Finally, my work has implications for further understanding the terrestrial glacial hydrology system, with implications for the dynamics of glaciers and glacial drainage under a warming climate. Indeed, advancing our understanding of the relationship between glacial hydrology and glacial dynamics process and form is also a state of the art topic for the interpretation of terrestrial glacial landscapes. For example, my recent fieldwork in Greenland’s west coast aims to identify interconnected subglacial cavities on the landscape surrounding Greenland’s fjords, and is inspired by similar martian landform identification strategies I applied for my work during this project.
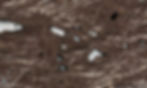
Shallow depressions, possible record of interlinked subglacial cavities on Western Greenland.